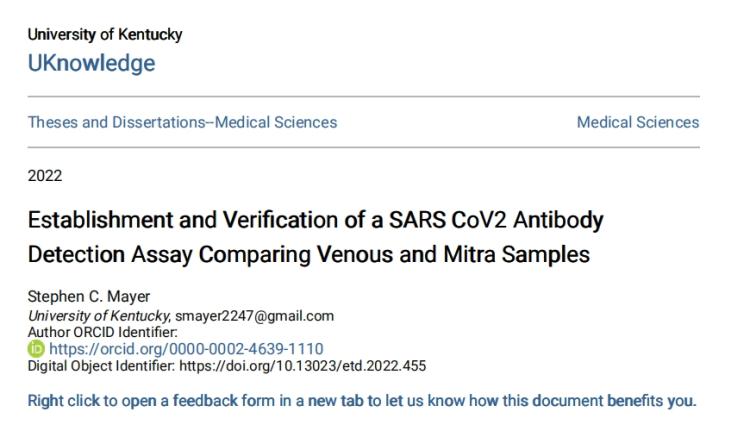
University of Kentucky
Theses and Dissertations--Medical Sciences
2022
Establishment and Verification of a SARS CoV2 Antibody Detection Assay Comparing Venous and Mitra Samples
Stephen C. Mayer
University of Kentucky, smayer2247@gmail.com
Author ORCID Identifier:
https://orcid.org/0000-0002-4639-1110
Digital Object Identifier: https://doi.org/10.13023/etd.2022.455
Recommended Citation
Mayer, Stephen C., "Establishment and Verification of a SARS CoV2 Antibody Detection Assay Comparing Venous and Mitra Samples" (2022). Theses and Dissertations--Medical Sciences. 24.
https://uknowledge.uky.edu/medsci_etds/24
This Master's Thesis is brought to you for free and open access by the Medical Sciences at UKnowledge. It has been accepted for inclusion in Theses and Dissertations--Medical Sciences by an authorized administrator of UKnowledge. For more information, please contact UKnowledge@lsv.uky.edu.
STUDENT AGREEMENT:
I represent that my thesis or dissertation and abstract are my original work. Proper attribution has been given to all outside sources. I understand that I am solely responsible for obtaining any needed copyright permissions. I have obtained needed written permission statement(s) from the owner(s) of each third-party copyrighted matter to be included in my work, allowing electronic distribution (if such use is not permitted by the fair use doctrine) which will be
submitted to UKnowledge as Additional File.
I hereby grant to The University of Kentucky and its agents the irrevocable, non-exclusive, and royalty-free license to archive and make accessible my work in whole or in part in all forms of media, now or hereafter known. I agree that the document mentioned above may be made
available immediately for worldwide access unless an embargo applies.
I retain all other ownership rights to the copyright of my work. I also retain the right to use in future works (such as articles or books) all or part of my work. I understand that I am free to register the copyright to my work.
REVIEW, APPROVAL AND ACCEPTANCE
The document mentioned above has been reviewed and accepted by the student’s advisor, on behalf of the advisory committee, and by the Director of Graduate Studies (DGS), on behalf of
the program; we verify that this is the final, approved version of the student’s thesis including all changes required by the advisory committee. The undersigned agree to abide by the statements above.
Stephen C. Mayer, Student
Dr. Jerold Woodward, Major Professor
Dr. Melinda Wilson, Director of Graduate Studies
Establishment and Verification of a SARS CoV2 Antibody Detection Assay Comparing
Venous and Mitra Samples
THESIS
A thesis submitted in partial fulfillment of the
requirements for the degree of Master of Science in the
College of Medicine
at the University of Kentucky
By
Stephen C. Mayer
Lexington, Kentucky
Director: Dr. Jerold Woodward, Professor of Immunology
Lexington, Kentucky
2022
Copyright © Stephen C. Mayer 2022
https://orcid.org/0000-0002-4639- 1110
ABSTRACT OF THESIS
Establishment and Verification of a SARS CoV2 Antibody Detection Assay Comparing Venous and Mitra Samples
During the COVID- 19 pandemic in 2020, the need for highly-specific, wide-spread, and
rapid serological testing surged. In this study, we showed very strong positive correlation
between venous blood collection and Mitra home sampling kits for the detection of anti-
spike IgGs using an in-house ELISA protocol (based on a protocol from Mt. Sinai). This
study demonstrates the utility of using at-home, patient-centric testing to enhance the
sero-surveillance methods currently in place for viral tracking and monitoring.
KEYWORDS: COVID- 19, Serological Testing, ELISA, SARS CoV2, Mitra
Stephen C. Mayer
(Name of Student)
11/25/2022
Date
Establishment and Verification of a SARS CoV2 Antibody Detection Assay ComparingVenous and Mitra Samples
By
Stephen C. Mayer
Dr. Jerold Woodward
Director of Thesis
Dr. Melinda Wilson
Director of Graduate Studies
11/25/2022
Date
ACKNOWLEDGMENTS
My most thanks to my thesis mentor and PI, Dr. Jerold Woodward, for his incredible wisdom and constant feedback for the entire duration of my time at the University of Kentucky. I would like to thank Dr. Donald Cohen for serving as a member of my defense committee and offering great tips and insight as he worked alongside myselffrequently in the laboratory. I would like to thank Dr. John Yannelli for being an incredible professor over two semesters, sparking my interest in translational medicine, and serving as a member of my defense committee. In addition, I would like to acknowledge Dr. Siva Gandhapudi, Mr. Martin. Ward, Mrs. Allison Sims, and Mr. John Peyton for supporting me in the laboratory and offering great feedback. Finally, I would like to thank JenniferMoylan of the Center for Clinical and Translational Sciences (CCTS) for helping to design this study, co-writing theIRB protocol, and recruiting subjects. This study was funded by
a CCTS pilot award to Dr. Jerold Woodward.
TABLE OF CONTENTS
CHAPTER 1. INTRODUCTION .......................................................................... 1
CHAPTER 2. MATERIALS AND METHODS .................................................... 8
2.1 Safety/Protocol Statement................................................................... 8
2.2 Participants and Sample Collection ................................................... 8
2.5 ELISA Plate Preparation .................................................................. 10
2.6 ELISA Plate Blocking ....................................................................... 11
2.7 Sample Preparation/Loading............................................................. 11
2.8 Detection Antibody Loading ............................................................. 12
2.9 ELISA Plate Development and Reading ........................................... 12
3.2 Antibody Titers of Positive and Negative Subjects ......................... 15
3.3 VAMS Antibody Stability at Ambient Temperature ......................... 16
LIST OF TABLES
LIST OF FIGURES
Figure 1A Reciprocal Serum Dilutions 1 of 3 .................................................... 17
Figure 1B: Reciprocal Serum Dilutions 2 of 3 ................................................... 18
Figure 1C: Reciprocal Serum Dilutions 3 of 3 ................................................... 19
Figure 2: Anti-spike RBD standard absorbance curves ...................................... 20
Figure 3A: Positive Control Reciprocal Serum Dilutions .................................. 21
Figure 3B: Negative Control Reciprocal Serum Dilutions ................................. 22
Figure 4: Correlation of Positive Sample OD Values at a 1:100 Dilution............ 23
CHAPTER 1. INTRODUCTION
During 2020, the rise of Severe Acute Respiratory Syndrome Coronavirus 2
(SARS CoV2), a virus that causes COVID- 19, brought great harm and destruction to the
human population, killing 6.5 million people and rising worldwide1. According to the
American Pandemic Preparedness Team, it is estimated that we will likely see an even
stronger pandemic within the next decade and more to come after2. To ensure early
detection and prevention of future pandemics, real-time population monitoring is
required, including tracking viral variants, viral-infection monitoring, and epidemic
analysis and forecasting. To do this successfully, highly sensitive serological testing must
be readily available and performed at a rapid rate. In regards to controlling active
pandemics, widespread epidemiological testing is needed in order to define disease
control methods in real-time and to make data-driven policy changes3.
Coronaviruses (CoV), varying in size from 65 to 125 nm, are the largest RNA
viruses currently known4. They are members of the genus Coronaviridae, pleomorphic
RNA based viruses that are composed of crown-shaped peplomers5. SARS CoV2 shares
considerable sequence homology with SARS-Coronavirus and the Middle East MERS-
Coronavirus and is a rapidly spreading respiratory virus worldwide, originally defined in
Wuhan, China5. Coronaviruses are classified based on the crown-like spikes present on
their outer membrane into one of four main categories, alpha (α -CoV), beta (β-CoV),
gamma (γ-CoV),or delta (δ-CoV)5. SARS-CoV-2 is classified into the β-CoV category
due to it being composed of positive-strand RNA with a lipid envelop and being able to
infect mammals6. Viral spike proteins are made up of two smaller subunits, S1 and S2. S1
protein is responsible for receptor binding as it contains the receptor binding domain
(RBD) and S2 contains the fusion machinery responsible for integrating the viral RNA
into the host cell membrane8.
SARS CoV2 human-human transmission occurs through aerial droplets during conversation, breathing, sneezing, or coughing when two people are nearby5. During an active infection, SARS CoV2 circulates around the body to find healthy host cells. The spike protein on the outer membrane of the viral surface binds to its cognate receptor on
the host cell, angiotensin-converting enzyme 2 (ACE2)5,7 . ACE2 contains 805 amino
acids. It is ubiquitous within the human body, but is overexpressed on cellular outer
membranes of the intestine, heart, lungs, kidney, testis, and brain8. Due to SARS CoV2 spreading as aerial droplets, virus enters the human body through the upper respiratory tract’s epithelial tissues and proceeds to the lower respiratory tract to infect alveolar and
bronchial epithelium, cells that also overexpress ACE28.
After ACE2 binding, the host cell’s transmembrane protease serine 2 (TMPRSS2)
cleaves the S1 and S2 subunits of the viral spike protein to allow fusion of the virus
envelope to the host cell’s plasma membrane and release of viral RNA into the cytosol7. The positive-strand RNA uses the host cell’s protein synthesis machinery to create viral
RNA polymerase and non-structural proteins needed for RNA replication7. The
subsequent products of RNA replication and transcription are the protein subunits needed
to construct more SARS CoV2 structures, including spike, envelope, membrane, and
nucleocapsid proteins. The structural and accessory proteins are then transported to the
Endoplasmic-Reticulum Golgi Intermediate Compartment (ERGIC), where they are
packaged into virions to be secreted back into the body’s circulation to infect other host
cells7.
As the infection progresses and epithelial cells are damaged and lysed by the
virus, the immune system begins to rapidly respond9. Due to this rapid response, innate
immune cells overproduce pro-inflammatory cytokines (IL-6, IL- 1β, TNF), small
proteins that can have paracrine, autocrine, or endocrine functions on other immune
cells10. When maintained within proper range, these cytokines are crucial for proper
immune system function and coordination. The acute rise of pro-inflammatory cytokines during an active SARS CoV2 infection results in the rapid influx of immune cells to the
site of infection, leading to damaged tissues and respiratory distress 10. Early detection
and proper treatment of this so called “cytokine storm” can lead to better clinical
outcomes for SARS CoV2 patients.
In order to effectively defeat the SARS CoV2 virus and limit human-human
transmission, mechanisms of host cell entry or viral replication must be prevented.
Coronaviruses and their possible vaccines have been studied for many years prior to the
SARS CoV2 pandemic in 202011. In 1984,a group at Harvard University in the United
States synthesized RNA polymerase enzymes to make synthetic messenger RNA
(mRNA) in the laboratory5. This was a major break-through in studying gene activity and
function. In the 1990s, synthetic mRNA was used as a treatment in rats for cancer and
influenza, but it was not until 2005 when mRNA was able to be genetically modified to be more stable in humans, leading to increased federal funding and widespread mRNA
vaccine research11.
The first two mRNA-based vaccines FDA-approved for large-scale use by
humans were for SARS CoV2 in 2020, produced by Pfizer-BioNTech and Moderna11.
There are many public skeptics and a number of conspiracy theories concerning RNA-
based vaccines that arose in response to the rapid timeline of vaccine development,
however, scientists and researchers were able to use the years of prior MERS-CoV and SARS-CoV vaccine research to adapt protocols to the SARS CoV2 virus and produce an
effective vaccine to curb transmission rates11,12 .
To develop effective mRNA-based vaccines, researchers exploited the virus’
need to bind to ACE2, with the goal of preventing viral entry into the host cell13. To do
this, the mRNA sequence of the SARS CoV2 spike protein was isolated in a laboratory
and packaged into a lipid nanoparticle13. Putting the recombinant mRNA spike protein
sequence inside of a lipid nanoparticle helps better stimulate the innate immune system’s professional antigen presenting cells (APCs) and prevents proteolysis of mRNA in vivo14.
After fusion of the lipid nanoparticle to the host cell membrane, the mRNA sequence is
taken up and transported to the ribosome for translation13. The subsequently produced
spike protein molecules will then be presented on the host cell’s surface by major
histocompatibility complexes (MHCs) for recognition by T cells. MHCs arepresent on
almost every cell inside the human body and are critically important for presenting
intracellular and extracellular peptides for immune-surveillance, determining if cells are healthy or need to be destroyed15. MHCs are highly variant and extremely difficult for
pathogens to evade due to their polygenic (high number of genes) and polymorphic
(multiple variants within a population) features 16.
After vaccination or viral infection, viral proteins arepresented by APCs in
peripheral lymphoid tissue. Following innate immune cell stimulation and expression of co-stimulatory molecules, CD4+ or CD8+ T-cells differentiate to carry out their effector
functions specific to spike protein or other viral proteins 16. In peripheral lymphoid tissues
(lymph nodes and spleen), B-cells also encounter spike protein antigens to become
activated with the help of follicular dendritic cells and TFH cells. B-cells can then
differentiate into either plasma B-cells to rapidly produce high-affinity antibodies
(Immunoglobulins, Ig) or memory B cells, enabling a morerapid response for future
infections16,17 . Antibodies can be separated into five classes based on their constant
regions and effector functions--IgM, IgD, IgG, IgA, and IgE. IgG antibodies have several
subclasses and is the most abundant isotype circulating in serum18. The newly
synthesized IgG spike protein-specific antibodies from the plasma B-cells help neutralize
SARS CoV2 by binding to spike protein molecules and blocking the interaction with
ACE2 on the host cell13. In addition, antibodies bound to pathogens enhance the binding of phagocytic immune cells to neutralized virions to help clear and dispose of the virus. Phagocytic immune cells (neutrophils and monocytes) contain Fc receptors that enable
binding to IgG antibody constant regions, thus promoting uptake of Ab-coated virus17.
The presence of spike protein antibodies in serum are one of the biological
markers that can help determine if a patient has been recently infected with SARS CoV2. To detect and quantify the presence of spike protein antibodies in serum, serological tests
such as an enzyme-linked immunosorbent assay (ELISA) can be conducted.
The development of the ELISA was a major breakthrough for the field of
Immunology, simultaneously invented by Dr. Perlam and Dr. Schuurs through
modifications of the existing radioimmunoassay (RIA)19. ELISAs are highly sensitive
serological tests that can be used to quantify small substances such as proteins, antigens, antibodies, hormones, and glycoproteins using very small sample volumes19. Due to their
high sensitivity, signal amplification, low cost, and potential for automation, ELISAs
have broad use in immunology research and diagnostics20. ELISA plates are made of
polystyrene and contain a certain number of wells that bind protein molecules with high- affinity. In regards to screening antibody levels in serum, an indirect ELISA can be used. Using this example, each well is coated with recombinant target protein and then blocked
with an agent (such as ovalbumin, BSA, or aprotinin) to prevent the binding and
interaction of any unwanted antibodies19. Unknown serum samples then can be placed
into the wells and if the serum contains antibodies specific to the coated protein of
interest, they will bind with high-affinity. Following awash-step, an enzyme-conjugated
secondary antibody is placed into the wells to allow interaction with any bound
antibody19. The addition of a substrate that interacts with the conjugated enzyme causes a
solution color change in the wells based on the amount of enzyme present, directly
correlating to the amount of bound primary antibody 19. Using a spectrophotometer,
ELISA plates can be read and quantified to give highly-accurate antibody titer levels for
unknown serum samples.
During the early phases of 2020, delayed diagnostic pipelines and asymptomatic SARS CoV2 infections were negatively affecting the ability to determine viral prevalence rates in Kentucky and around the World21. The need for highly-specific, wide-spread, and rapid serological testing surged. Conventional serological sample collection methods that require venous phlebotomy from patients, especially in an uncontrolled pandemic, do not
effectively meet the demand for urgency. Rapid testing and properly defined viral
prevalence rates allow experts to monitor trends of infection in the general population,
stop geographical spread, and determine potential associated risk factors of the disease22.
Major rate limiting steps must be addressed with conventional serological sample
collection protocols to better respond to future pandemics and diseases. Currently,
limitations of high-scale serological testing include the need for a large number of
healthcare professionalstrained in venipuncture, patients having to schedule
appointments and come into clinics, proper sample shipping, and the cost of labor needed
in a research laboratory to properly process and store samples for testing23. In
rural/remote areas affected by healthcare disparities, limitations with collection and
testing can widen these disparities even further during an active pandemic.
In the early stages of a pandemic, it is important for people to stay at home and
limit contact with others as much as possible to decrease the spread of disease. The
development of highly sensitive mail-in sampling kits and serological assays would
relieve the pressure on healthcare facilities and increase access to testing, not only for
SARS CoV2, but any future virus. Using a Mitra microsampler, with Neotryx’s
volumetric absorptive microsampling (VAMS) technology, we tested if anti-spike IgG
antibody titers of capillary blood samples were comparable to IgG antibody titers from
venous blood samples. Comparable results would remove the need for venous blood
sample collection, have major implications for how we manage the rise of future
pandemics, and will help enhance population disease serosurveillance protocols currently
in place.
CHAPTER 2. MATERIALS AND METHODS
2.1 Safety/Protocol Statement
This study was performed under an approved IRB protocol: #60876, Covid- 19 Antibodies in Finger Stick Capillary Samples. Biosafety approval was obtained from
the University of Kentucky’s Institutional Biosafety Committee to work with blood
samples from positive COVID- 19 patients. In order to protect laboratory staff, proper
PPE (lab coats, protective eyewear, disposable gloves) were worn, blood was
centrifuged using rotor buckets with caps, and all samples were manipulated in a
certified Biosafety Cabinet prior to heat inactivation.
2.2 Participants and Sample Collection
20 volunteers from Kentucky, varying in age, were recruited for this study and
divided into two groups. Group 1 was composed of 10 healthy participants that
previously received a positive test for SARS CoV2 (i.e. recovered) and group 2 was
composed of 10 healthy participants with no known previous exposure or symptoms. The
prior SARS CoV2 infection status was self-reported. None of the subjects were
vaccinated. Subjects were de-identified and assigned a sample number depending on their
group (FS-01 - FS- 10 in group 1 and FS- 11 - FS-20 in group 2).
Subjects were scheduled for an outpatient venous and capillary phlebotomy
appointment with a clinician at the University of Kentucky’s Center for Clinical and
Translational Science. Prior to the appointment, subjects were tested for an active SARS CoV 2 infection via PCR and it was required that the negative control group produce a
test negative.
Venous blood samples were collected into a 4 mL BD vacutainer CPT tube.
Capillary blood samples were collected using VAMS (Mitra Collection Kit; Neoteryx, CA, USA). To obtain capillary blood, a contact-activated lancet was used to prick the subject’s fingertip. Approx. 20 uL of capillary blood was absorbed onto each Mitra tip,
collecting two in total.
2.3 Sample Storage
Upon collection, venous and capillary samples were sent directly to the Human
Immune Monitoring Lab (Biosafety-Level 2+) where they were immediately processed at room temperature (20-25oC). Venous blood sample CPT vacutainers were centrifuged for
30 minutes at 2850 rpm (brakes disengaged) to separate the serum and red blood cells.
After centrifugation, serum plasma was extracted from the top layer of the vacutainer anddispensed into 5 ml FACS culture vials and heat-inactivated at 56oC for 30 min. Serum was then transferred into freezer vials and stored at -80oC until needed for ELISA assay. Capillary blood samples remained dehydrated on Mitra tips in sealed packages at ambient
temperature until needed for testing.
2.4 Mitra Tip Hydration
One day prior to the ELISA, capillary Mitra tips were placed into 1 ml
microcentrifuge tubes containing 200 uL of 1X PBS (ensuring each tip was fully
submerged) and left to hydrate overnight at 4oC. On the day of the ELISA, capillary
VAMS samples were removed from storage and directly used in subsequent ELISA
assays (assuming a starting dilution of 1:10, 20 uL of dehydrated blood into 200 uL of 1X
PBS). Any hydrated samples not used in testing were placed at -80oC until needed
further.
2.5 ELISA Plate Preparation
One day prior to running the ELISA, all plates were coated with recombinant
SARS CoV2 full-length spike protein in preparation for the assay. Spike protein was
diluted to 2 ug/ml from 2 mg/ml stock solution with 1X PBS. 50 ul of 2 ug/ml spike was
added to each well of a clear flat-bottom immuno nonsterile 96-well plate [Immulon
4HBX Thermo Scientific ™ Cat # 3855 or equivalent] (ensuring the bottoms were
completely covered in solution). Plates were then covered with plastic and stored at 4oC
overnight to allow for protein binding.
2.6 ELISA Plate Blocking
On the day of the ELISA, the plates were removed from storage and were washed
with 1X PBS 0.1% tween 20 three times at 200 ul/well using an Aquamax 2000 plate
washer.
After washing, any remaining wash solution was discarded and 200 ul of 3%
(w/v) non-fat dry milk in 1X PBS 0.1% tween buffer (Omniblok™ non-fat dry milk from
Americanbio Inc; Cat # AB1010901000) was added to each well for blocking. Plates
were then incubated at ambient temperature for 1 hour.
2.7 Sample Preparation/Loading
Capillary VAMS tips were removed from 4oC storage and serum samples from - 80oC to let thaw. Once serum was thawed, a 1:10 dilution in 1X PBS was made for each sample. To prepare the standard curve, a 25 ug/ml dilution of Genscript anti-spike RBD
(HC2001, monoclonall antibody) in 1X PBS was made.
After discarding the blocking buffer, 120 ul of 1% (w/v) non-fat dry milk in 1X PBS 0.1% tween buffer was added to all wells with an additional 42 ul in the top row of each plate. Samples were then loaded in duplicates in the top row of the plates adding 18
ul /well of STD curve anti-spike RBD (25 ug/ml), 1:10 serum samples, and 1:10 Mitra
samples. Using a multichannel pipette, the toprows were mixed thoroughly and 60 ul
was then transferred down to the next row, repeating this process for 7 rows and
discarding the final 60 ul from row 7. Row 8 was left blank. Plates were incubated at
ambient temperature for 1 hour to allow for primary antibody-protein binding.
2.8 Detection Antibody Loading
Plates were washed with 1X PBS 0.1% tween 20 three times at 200 ul/well using
an Aquamax 2000 plate washer. A 1:1500 dilution of Goat anti-mouse IgG-HRP (50%
glycerol stock Jackson Immunoresearch, 115035003) was made with 1% (w/v) non-fat
dry milk in 1X PBS 0.1% tween buffer. 50 ul/well was then added to each plate and
incubated at ambient temperature for 1 hour to allow for secondary antibody-primary IgG
antibody binding.
2.9 ELISA Plate Development and Reading
Plates were washed with 1X PBS 0.1% tween 20 three times at 200 ul/well using
an Aquamax 2000 plate washer. HRP-substrate (SIGMAFAST™ OPD Sigma-Aldrich:
Cat#P9187 or equivalent) was made by dissolving one urea hydrogen peroxide tablet into
20 ml of dH2O. Once dissolved, an OPD (0-phenylenediamine dihydrochloride) tablet
was added to the solution and vortexed. After discarding any remaining wash solution in the plates, 100 ul of the OPD solution was added to all wells of each plate and developed at ambient temperature for 10 min. After 10 min, 50 ul/well of 3.0 M Hydrochloric Acid
(Fisher Scientific: Cat#S25856, or equivalent) was added to stop the enzyme-substrate
reaction. Plates were then immediately scanned andread at a 490 nm wavelength on a
spectrophotometer.
2.10 Data Analysis
For each plate, the average of the negative controls (blank OD values) plus three
times the standard deviation of the mean determined the level of background for each
plate. Any sample with an optical density value 2X above the calculated background was considered positive for anti-spike IgG. Venous and capillary VAMS antibody titer curves
were plotted in duplicates for comparison. Correlation analysis was conducted by
averaging and plotting the optical density duplicates of each positive sample at a 1:100 serum dilution factor. As a positive control to ensure each ELISA was performed under
optimal conditions, a standard curve using anti-spike RBD monoclonal antibody at a
known concentration (25 ug/ml) was established for each plate.
CHAPTER 3. RESULTS
3.1 Agreement of Capillary VAMS and Venous Anti-Spike IgG Antibody Titers
It has been demonstrated previously that capillary blood sampling shows major promise for the future of serological testing21,23,24 . In support of these findings, our study
demonstrated the utility of capillary blood sampling with the development of a highly
qualitative and quantitative SARS CoV2 ELISA protocol (based on the Mt. Sinai SARS
CoV2 ELISA protocol25).
To determine the correlation of the reciprocal serum dilution curves between
capillary VAMS and venous sampling, all OD values were graphed in duplicates (Figures
1A, 1B, and 1C). For better graphical visualization of sample correlation, subjects were
separated based on whether their max OD reading was high (>1.5), medium (>0.5), or
low (<0.5). There was excellent agreement of the dilution curves between venous and
Mitra sampling for all levels of positivity. Importantly, we performed the comparison of venous and Mitra sampling for a particular individual within the same ELISA assay. A standard curve for every plate of each ELISA was produced by plotting the OD values of the positive control (anti-RBD) (Figure 2). For those assays using multiple plates, there
was excellent agreement of the standard curve between plates, indicating that all intra-
assay comparisons were valid.
To determine the degree of correlation between venous and Mitra sampling, we
compared anti-spike IgG optical density absorbance values in capillary VAMS and
venous samples. We averaged the duplicates of each positive sample at a 1:100 dilution
and plotted the data on a scatter plot. Using 13 positive test subjects, we found strong
correlation between the two sampling methods (R= 0.985) (Figure 4). These results
provide a strong statistical demonstration of the correlation between venous and Mitra
sampling for the measurement of anti-spike IgG antibody.
3.2 Antibody Titers of Positive and Negative Subjects.
Antibody titers were calculated based on the serum dilution curves shown in
Figures 1A, 1B, and 1C. We separated the titers of the 20 subjects into two groups. Group
1 was composed of 10 healthy participants that previously received a positive test for
SARS CoV2 (i.e., recovered) (self-reported) and group 2 was composed of 10 healthy
participants with no known previous exposure or symptoms. Three out of 10 subjects in
group 1 showed a strong titer consistent with prior SARS CoV2 infection while 7/10
showed a weak titer from both venous and Mitra samples (Figure 3A). Overall, the titers of group 1 were significantly higher than those of group two (Figure 3B), consistent with
their SARS CoV2 negative status. Out of the 9 tested subjects in group 1, all 9 had OD
values above the assigned background for that assay, meaning they were positive for
serum anti-spike IgG antibodies. Out of 9 subjects in the negative control group, 3 had
OD values above background while 6 did not meet the assay positivity threshold. We
cannot exclude that these three subjects had a prior asymptomatic SARS CoV2 infection.
3.3 VAMS Antibody Stability at Ambient Temperature
Prior studies have shown antibody stability on DBS filter paper for more than 6
months, but dueto VAMS being a newer sampling system, antibody stability is still being tested26. Upon receiving the dehydrated capillary VAMS samples from the University of
Kentucky’s Center for Clinical and Translational Science, we stored them at ambient
temperature sealed in zip-lock bags. Due to receiving the samples ahead of when the
SARS CoV2 assay was optimized, they were stored for varying number of days before being hydrated for use (Table I). While our original intent of the project did not include
testing antibody stability, the results of our study show that antibodies were stable on
Neoteryx’s capillary VAMS kits dehydrated at ambient temperature for up to 126 days. Capillary VAMS antibody stability is proven through the IgG titer positive correlation
with venous sampling shown in Figure 4.
Figure 1A Reciprocal Serum Dilutions 1 of 3. Paired serum (S) (circles) and capillary VAMS (M) (triangles) from 20 subjects were compared in a SARS CoV-2 ELISA
measuring serum spike IgG antibody concentrations. OD values (read at 490 nm) were plotted with their corresponding reciprocal serum dilution to create a spike IgG dilution curve. The number listed next to each sample represents the ELISA assay they were
tested in and their corresponding standard curves can be found in Figure 2. The suffix after the subject number denotes whether they were previously positive (P) or negative (N) for SARS CoV2.
Figure 1B: Reciprocal Serum Dilutions 2 of 3.
Figure 1C: Reciprocal Serum Dilutions 3 of 3.
Figure 2: Anti-spike RBD standard absorbance curves. For every ELISA (E35, E36,
E44, E45, and E48) standard curves were produced for each plate at a concentration of 25 ul/ml. Using the standard curve, you can predict the serum IgG concentrations in an
unknown sample and it shows you the maximum OD value for any given assay. A new standard curve was produced for each assay to ensure reliable data interpretation.
Figure 3A: Positive Control Reciprocal Serum Dilutions. All positive control
individuals (FS-01 thru FS- 10) were plotted with their corresponding reciprocal serum dilutions. Paired serum is represented as circles and capillary VAMS represented with triangles. FS-06 omitted due to improper capillary VAMS sampling. FS-02, FS-04, FS- 07, and FS- 10 showed very high concentrations of anti-spike IgG in serum, but all
subjects in the positive control group met their assay positivity threshold.
Figure 3B: Negative Control Reciprocal Serum Dilutions. All negative control
individuals (FS- 10 thru FS-20) were plotted with their corresponding reciprocal serum dilutions. Paired serum is represented as circles and capillary VAMS represented with triangles. FS- 12 omitted due to improper capillary VAMS sampling. While the negative control group’s reciprocal serum dilutions are much lower than those of the positive
control group, FS- 13, FS- 14, and FS-20 met the positivity threshold for their given ELISA.
Figure 4: Correlation of Positive Sample OD Values at a 1:100 Dilution. To
determine if capillary VAMS and venous serum anti-spike IgG concentrations correlate, we can take the average of the OD duplicates for each positive sample at a given dilution
factor and plot them. OD values directly correlate with anti-spike IgG serum
concentration levels. A strong positive correlation between the two testing methods is shown (R=0.985).
Table 1:Capillary VAMS pre-hydration storage times. For each test subject, the date of collection represents the date the University of Kentucky’s Human Immune
Monitoring Lab received the capillary samples from the Center for Clinical and
Translational Science and the date of hydration represents the day they were hydrated in 1X PBS.
CHAPTER 4. DISCUSSION
Especially in the early phases of a pandemic, it is critical to understand disease
seroprevalence within a population to better determine disease control methods and stop
geographical spread. Our results demonstrate that there is a very strong positive
correlation between venous blood collection and Mitra home sampling for the detection
of anti-spike IgGs. During the course of our study, other labs in the U.S. were
concurrently testing capillary blood sampling to determine if this method would be
acceptable for managing and monitoring the rise of future diseases. All of these groups
showed similar results between the two sampling methods23,24,25 . Addingto this research,
our lab further demonstrated serum antibody correlation of capillary VAMS and
conventional venous sampling methods using a highly-specific and quantifiable SARS
CoV2 IgG enzyme-linked immunosorbent assay (Figure 4).
Due to limited serosurveillance protocols and improper testing methods at the
start of the COVID- 19 pandemic, many asymptomatic cases went undetected, causing the
seroprevalence rates to be much higher than officials were estimating27. Subjects from
our negative control group, FS- 13, FS- 14, and FS-20, had anti-spike IgG serum
concentrations that were above the positivity threshold of the ELISA, implicating that
they could have been asymptomatic carriers of SARS CoV2, as they had no known
symptoms or positive test prior to the study. Using Figure 3B for reference, the three
sero-positive subjects’ endpoint titers were much lower than the positive subjects in
Figure 3A. Eventhough they met the positivity threshold of the ELISA, their anti-spike IgG serum concentrations were still very low, meaning that if they were an asymptomatic
case of SARS CoV2, their immune response was not robust enough to produce high
concentrations of serum anti-spike IgGs. In Figure 3A, endpoint titers of IgGs were much
higher, meaning their immune response during an active infection was more profound
and produced high levels of anti-spike IgG. While this assay has a high sensitivity and
accuracy for detecting spike-specific IgGs in serum, we cannot conclude that those who
meet the positivity threshold or a certain concentration threshold will have protective
immunity toward a future SARS CoV2 infection. IgG viral neutralization effects vary by
individual based on the quantity and time of SARS CoV2 exposure and their body’s
overall immune response28. We can assume that as a patient’s serum anti-spike IgG
concentration increases, they would have a better ability to neutralize SARS CoV2, but the neutralizing effects of anti-spike IgG have been shown to decline rapidly 5-8 weeks
post-infection, but can remain detectable for up to 8 months29.
Although not originally in the study design, we also demonstrated antibody
stability for up to 126 days at ambient temperature when dehydrated on Neoteryx’s
VAMS collection swab. In a publication by the National Institutes of Health studying
capillary VAMS antibody stability, a research group showed 94% stability up to 21 days
at room temperature before placing the samples at -20oC24. Our study further adds to
these findings and increases the utility of capillary VAMS collection kits due to not
needing any special storage or shipping protocols.
Using this minimally invasive and quick method of testing will allow kits to be
sent in large numbers to people around the world, including rural and remote locations,
allow for an exponential decrease in sample processing time, and reduce the cost
associated with venous sampling and testing. Our highly-sensitive and quantitative SARS
CoV2 ELISA protocol allows, with minor modifications, for use with virtually any viral
infection arising in the future. In combination with mail-in blood sampling kits, the way
we monitor and control diseases within a population will forever change.
REFERENCES
1. World Health Organization. (n.d.). Who coronavirus (COVID-19)
dashboard. World Health Organization. Retrieved September 1, 2022, from https://covid19.who.int/
2. American pandemic preparedness: Transforming our capabilities - white house. (n.d.). Retrieved September 3, 2022, from
https://www.whitehouse.gov/wp-content/uploads/2021/09/American-Pandemic- Preparedness-Transforming-Our-Capabilities-Final-For-Web.pdf?page=29
3. Kelly-Cirino, C. D., Nkengasong, J., Kettler, H., Tongio, I., Gay-Andrieu, F., Escadafal, C., Piot, P., Peeling, R. W., Gadde, R., & Boehme, C. (2019).
Importance of diagnostics in epidemic and pandemic preparedness. BMJ global health, 4(Suppl 2), e001179. https://doi.org/10.1136/bmjgh-2018-001179
4. Bchetnia, M., Girard, C., Duchaine, C., & Laprise, C. (2020). The
outbreak of the novel severe acute respiratory syndrome coronavirus 2 (SARS- CoV-2): A review of the current global status. Journal of infection and public health, 13(11), 1601- 1610. https://doi.org/10.1016/j.jiph.2020.07.011
5. Muralidar, S., Ambi, S. V., Sekaran, S., & Krishnan, U. M. (2020). The
emergence of COVID- 19 as a global pandemic: Understanding the epidemiology, immune response and potential therapeutic targets of SARS-CoV-2. Biochimie,
179, 85- 100. https://doi.org/10.1016/j.biochi.2020.09.018
6. Wartecki A, Rzymski P. On the Coronaviruses and Their Associations with the Aquatic Environment and Wastewater. Water. 2020; 12(6):1598.
https://doi.org/10.3390/w12061598
7. Harrison, A. G., Lin, T., & Wang, P. (2020). Mechanisms of SARS-CoV- 2 Transmission and Pathogenesis. Trends in immunology, 41(12), 1100- 1115.
https://doi.org/10.1016/j.it.2020.10.004
8. Shirbhate, E., Pandey, J., Patel, V. K., Kamal, M., Jawaid, T., Gorain, B., Kesharwani, P., & Rajak, H. (2021). Understanding the role of ACE-2 receptor in pathogenesis of COVID- 19 disease: a potential approach for therapeutic
intervention. Pharmacological reports : PR, 73(6), 1539- 1550. https://doi.org/10.1007/s43440-021-00303-6
9. Rauti, R., Shahoha, M., Leichtmann-Bardoogo, Y., Nasser, R., Paz, E.,
Tamir, R., Miller, V., Babich, T., Shaked, K., Ehrlich, A., Ioannidis, K., Nahmias, Y., Sharan, R., Ashery, U., & Maoz, B. M. (2021). Effect of SARS-CoV-2
proteins on vascular permeability. eLife, 10, e69314.
https://doi.org/10.7554/eLife.69314
10. Ragab, D., Salah Eldin, H., Taeimah, M., Khattab, R., & Salem, R. (2020). The COVID- 19 Cytokine Storm; What We Know So Far. Frontiers in
immunology, 11, 1446. https://doi.org/10.3389/fimmu.2020.01446
11. Mayo Foundation for Medical Education and Research. (n.d.). History of
covid-19: Outbreaks and vaccine timeline. Mayo Clinic. Retrieved September 5, 2022, from https://www.mayoclinic.org/coronavirus-covid- 19/history-disease- outbreaks-vaccine-timeline/covid- 19
12. Levin, J., Bradshaw, M. Determinants of COVID- 19 skepticism and SARS- CoV-2 vaccine hesitancy: findings from a national population survey of U.S.
adults. BMC Public Health 22, 1047 (2022). https://doi.org/10.1186/s12889-022- 13477-2
13. Mascellino, M. T., Di Timoteo, F., De Angelis, M., & Oliva, A. (2021). Overview of the Main Anti-SARS-CoV-2 Vaccines: Mechanism of Action, Efficacy and Safety. Infection and drug resistance, 14, 3459–3476.
https://doi.org/10.2147/IDR.S315727
14. Hou, X., Zaks, T., Langer, R. et al. Lipid nanoparticles for mRNA delivery.
Nat Rev Mater 6, 1078– 1094 (2021).https://doi.org/10.1038/s41578-021-00358-0 15. Janeway CA Jr, Travers P, Walport M, et al. Immunobiology: The Immune
System in Health and Disease. 5th edition. New York: Garland Science; 2001.
The major histocompatibility complex and its functions. Available from:
https://www.ncbi.nlm.nih.gov/books/NBK27156/
16 Gulati, P. (2009), Janeway's Immunobiology, 7th Edition by Kenneth Murphy,
Paul Travers, and Mark Walport. Biochem. Mol. Biol. Educ., 37: 134-134.
https://doi.org/10.1002/bmb.20272
17 Dang, V. D., Hilgenberg, E., Ries, S., Shen, P., & Fillatreau, S. (2014). From the regulatory functions of B cells to the identification of cytokine-producing
plasma cell subsets. Current opinion in immunology, 28, 77–83.
https://doi.org/10.1016/j.coi.2014.02.009
18. Schroeder, H. W., Jr, & Cavacini, L. (2010). Structure and function of
immunoglobulins. The Journal of allergy and clinical immunology, 125(2 Suppl2), S41–S52.https://doi.org/10.1016/j.jaci.2009.09.046
19.Alhajj M, Farhana A. Enzyme Linked Immunosorbent Assay.[Updated 2022 Feb 2]. In: StatPearls [Internet]. Treasure Island (FL): StatPearls Publishing; 2022 Jan-. Available from:https://www.ncbi.nlm.nih.gov/books/NBK555922/
20. Arya, S. K., & Estrela, P. (2018). Recent Advances in Enhancement Strategies for Electrochemical ELISA-Based Immunoassays for Cancer Biomarker
Detection. Sensors (Basel, Switzerland), 18(7), 2010.
https://doi.org/10.3390/s18072010
21. Kalish, H., Klumpp-Thomas, C., Hunsberger, S., Baus, H. A., Fay, M. P., Siripong, N., Wang, J., Hicks, J., Mehalko, J., Travers, J., Drew, M., Pauly, K.,
Spathies, J., Ngo, T., Adusei, K. M., Karkanitsa, M., Croker, J. A., Li, Y.,
Graubard, B. I., … Sadtler, K. (2021, January 1). Mapping a pandemic: SARS-
COV-2 seropositivity in the United States. medRxiv. Retrieved October 11, 2022, from https://www.medrxiv.org/content/10.1101/2021.01.27.21250570v1.full-text 22. Kumar, M. S., Bhatnagar, T., Manickam, P., Kumar, V. S., Rade, K., Shah,
N., Kant, S., Babu, G. R., Zodpey, S., Girish Kumar, C. P., Vivian Thangaraj, J.
W., Chatterjee, P., Kanungo, S., Pandey, R. M., Murhekar, M., Singh, S. K.,
Sarkar, S., Muliyi, J. P., Gangakhedkar, R. R., & Reddy, D. (2020). National sero- surveillance to monitor the trend of SARS-CoV-2 infection transmission in India:
Protocol for community-based surveillance. The Indian journal of medical research, 151(5), 419–423.https://doi.org/10.4103/ijmr.IJMR 1818 20 23. Brown, L., Byrne, R. L., Fraser, A., Owen, S. I., Cubas-Atienzar, A. I.,
Williams, C. T., Kay, G. A., Cuevas, L. E., Fitchett, J., Fletcher, T., Garrod, G.,
Kontogianni, K., Krishna, S., Menzies, S., Planche, T., Sainter, C., Staines, H. M., Turtle, L., & Adams, E. R. (2021). Self-sampling of capillary blood for SARS-
CoV-2 serology. Scientific reports, 11(1), 7754.https://doi.org/10.1038/s41598- 021-86008-5
24. Wang, J., Li, D., Wiltse, A., Emo, J., Hilchey, S. P., & Zand, M. S. (2019). Application of volumetric absorptive microsampling (VAMS) to measure
multidimensional anti-influenza IgG antibodies by themPlex-Flu assay. Journal
of clinical and translational science, 3(6), 332–343.
https://doi.org/10.1017/cts.2019.410
25.Żak, M. M., Stock, A., Stadlbauer, D., Zhang, W., Cummings, K., Marsiglia, W., Zargarov, A., Amanat, F., Tamayo, M., Cordon-Cardo, C., Krammer, F., & Mendu, D. R. (2021). Development and characterization of a quantitative ELISA
to detect anti-SARS-CoV-2 spike antibodies. Heliyon, 7(12), e08444.
https://doi.org/10.1016/j.heliyon.2021.e08444
26. Vai, S., Cavallo, R., Angeretti, A., Ferrara, B., Bongiasca, G., Voglino, G., & Merlino, C. (1987). Stabilita degli anticorpi specificinel sangue essiccato su carta da filtro [Stability of specific antibodies in blood collected on filter paper disks]. Giornaledi batteriologia, virologia ed immunologia, 80(1- 12), 253–261.
27. Brown TS, Walensky RP. Serosurveillance and the COVID-19 Epidemic in
the US: Undetected, Uncertain, and Out of Control. JAMA. 2020;324(8):749–751. doi:10.1001/jama.2020.14017
28. Maeda, K., Higashi-Kuwata, N., Kinoshita, N. et al. Neutralization of SARS-
CoV-2 with IgG from COVID- 19-convalescent plasma. Sci Rep 11, 5563 (2021).
https://doi.org/10.1038/s41598-021-84733-5
29. Dispinseri, S., Secchi, M., Pirillo, M.F. et al. Neutralizing antibody responses to SARS-CoV-2 in symptomatic COVID- 19 is persistent and critical for survival. Nat Commun 12, 2670 (2021). https://doi.org/10.1038/s41467-021-22958-8
VITA
Stephen C. Mayer
Education
B.S. in Biology, May 2020 – Western Kentucky University’s Honors College
M.S. in Medical Sciences, expected Dec. 2022- University of Kentucky
Academic Positions
Biology Department Teaching Assistant, 2018-2020- Western Kentucky University
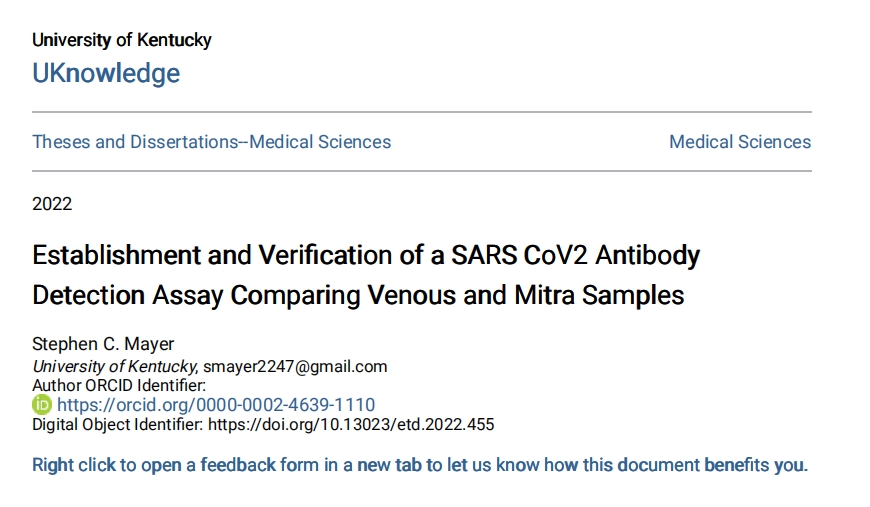